
Publications
A full list of publications can be found on Google Scholar
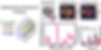
Oleic acid differentially affects lipid droplet storage of de novo synthesized lipids in hepatocytes and adipocytes
DOI: 10.1039/D3CC04829B
PMID: 37873279
[24] Castillo, H.B. †; Shuster, S.O. †; Tarekegn, L.H.; Davis, C.M. “Oleic acid differentially affects lipid droplet storage of de novo synthesized lipids in hepatocytes and adipocytes,” Chem. Comm. 2024, in press. DOI: 10.1101/2023.10.04.560581
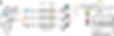
Site-specific crosslinking reveals phosphofructokinase-L inhibition drives self-assembly and attenuation of protein interactions
DOI: 10.1016/j.jbior.2023.100987
PMID: 38706136
[23] Sivadas, A.†; McDonald, E.F.†; Shuster, S.O.; Davis, C.M.; Plate, L. “Site-specific crosslinking reveals phosphofructokinase-L inhibition drives self-assembly and attenuation of protein interactions,” Adv. Biol. Regul. 2023, 90: 100987. DOI: 10.1016/j.jbior.2023.100987

Chemical interactions modulate λ6-85 stability in cells
DOI: 10.1002/pro.4698
PMID: 37313657
[22] Knab, E.; Davis, C.M. “Chemical interactions modulate λ6-85 stability in cells,” Protein Sci. 2023, 32 (7): e4698.DOI: 10.1002/pro.4698

Spatiotemporal heterogeneity of de novo lipogenesis in fixed and living single cells
PMID: 36976708
[21] Shuster, S.O.; Burke, M.J.; Davis, C.M. “Spatiotemporal heterogeneity of de novo lipogenesis in fixed and living single cells,” J. Phys. Chem. B, 2023, 127 (13): 2918-2926. DOI: 10.1021/acs.jpcb.2c08812

An in vitro cytomimetic of in-cell RNA folding
PMID: 35999178
[20] Yoo, H.; Davis, C.M. “An in vitro cytomimetic of in-cell RNA folding,” ChemBioChem. 2022, 23 (20): e202200406. DOI: 10.1002/cbic.202200406
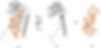
Spliceosomal SL1 RNA binding to U1-70k: the role of the extended RRM
DOI: 10.1093/nar/gkac599
PMID: 35876068
[19] Gopan, G.†; Ghaemi, Z.†; Davis, C.M.; Gruebele, M. “Spliceosomal SL1 RNA binding to U1-70K: the role of the extended RRM,” Nucl. Acids Res. 2022, 50 (14): 8193-8206. DOI: 10.1093/nar/gkac599
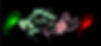
Cellular sticking can strongly reduce complex binding by speeding dissociation
PMID: 33826329
[18] Davis, C.M.*; Gruebele, M.* “Cellular sticking can strongly reduce complex binding by speeding dissociation,” J. Phys. Chem. B 2021, accepted. DOI: 10.1021/acs.jpcb.1c00950

Cytoskeletal drugs modulate off-target protein folding landscapes inside cells
DOI: 10.1021/acs.biochem.0c00299
PMID: 32567840
[17] Davis, C.M.*; Gruebele, M.* “Cytoskeletal drugs modulate off-target protein folding landscapes inside cells,” Biochemistry 2020, 59 (28), 2650-2659. DOI: 10.1021/acs.biochem.0c00299
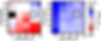
An in vitro mimic of in-cell solvation for protein folding studies
DOI: 10.1002/pro.3833
PMID: 31994240
[16] Davis, C.M.*; Deutsch, J.C.; Gruebele, M.* “An in vitro mimic of in-cell solvation for protein folding studies,” Protein Sci. 2020, 29 (4), 1046-1054.
Quantifying protein dynamics and stability in a living organism
DOI: 10.1038/s41467-019-09088-y
PMID: 30862837
[15] Feng, R.; Gruebele, M.*; Davis, C.M.*“Quantifying protein dynamics and stability in a living organism,” Nat. Commun. 2019, 10, 1179.

Cell volume controls protein stability and compactness of the unfolded state
PMID: 30289261
[14] Wang, Y.†; Sukenik, S.*†; Davis, C.M.; Gruebele, M.* “Cell volume controls protein stability and compactness of the unfolded state,” J. Phys. Chem. B 2018, 122 (49), 11762-11770.

A quantitative connection of experimental and simulated folding landscapes by vibrational spectroscopy
DOI: 10.1039/C8SC03786H
PMID: 30647892
[13] Davis, C.M.†; Polzi, L.Z.†; Gruebele, M.; Amadei, A.; Dyer, R.B.*; Daidone, I.* “A quantitative connection of experimental and simulated folding landscapes by vibrational spectroscopy,” Chem. Sci. 2018, 9, 9002-9011.
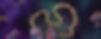
Soluble zwitterionic poly(sulfobetaine) destabilizes proteins
DOI: 10.1021/acs.biomac.8b01120
PMID: 30064224
[12] Kisley, L.; Serrano, K.M.; Davis, C.M.; Guin, D.; Murphy, E.; Gruebele, M.*; Leckband, D.E.* “Soluble zwitterionic poly(sulfobetaine) destabilizes proteins,” Biomacromolecules 2018, 19 (9), 3894-3901.
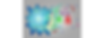
Non-steric interactions predict the trend and steric interactions the offset of protein stability in cells
PMID: 29877016
[11] Davis, C.M.; Gruebele, M. “Non-steric interactions predict the trend and steric interactions the offset of protein stability in cells,” ChemPhysChem 2018, 19 (18), 2290-2294.

Labeling for quantitative comparison of imaging measurements in vitro and in cells
DOI: 10.1021/acs.biochem.8b00141
PMID: 29546761
[10] Davis, C.M.*; Gruebele, M.* “Labeling for quantitative comparison of imaging measurements in vitro and in cells,” Biochemistry 2018, 57 (13), 1929-1938.
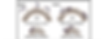
Binding, folding, and insertion of a β-hairpin peptide at a lipid bilayer surface: influence of electrostatics and lipid tail packing
DOI: 10.1016/j.bbamem.2017.12.019
PMID: 29291379
[9] Reid, K.; Davis, C.M.; Dyer, R.B.; Kindt, J.T. “Binding, folding, and insertion of a β-hairpin peptide at a lipid bilayer surface: influence of electrostatics and lipid tail packing,” Biochim. Biophys. Acta Biomembr. 2018, 1860 (3), 792-800.

How does solvation in the cell affect protein folding and binding?
DOI: 10.1016/j.sbi.2017.09.003
PMID: 29035742
[8] Davis, C.M.; Gruebele, M.; Sukenik, S. “How does solvation in the cell affect protein folding and binding?” Curr. Opin. Struct. Biol. 2018, 48, 23-29.
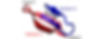
Parallel folding pathways of Fip35 WW domain explained by infrared spectra and their computer simulation
PMID: 28881468
[7] Polzi, L.Z.; Davis, C.M.; Gruebele, M.; Dyer, R.B.; Amadei, A.; Daidone, I. “Parallel folding pathways of Fip35 WW domain explained by infrared spectra and their computer simulation,” FEBS Lett. 2017, 591 (20), 3265-3275.

Dual time-resolved temperature-jump fluorescence and infrared spectroscopy for the study of fast protein dynamics
DOI: 10.1016/j.saa.2017.01.069
PMID: 28189834
[6] Davis, C.M.; Reddish, M.J.; Dyer, R.B. “Dual time-resolved temperature-jump fluorescence and infrared spectroscopy for the study of fast protein dynamics,” Spectrochim. Acta A 2017, 178, 185-191.
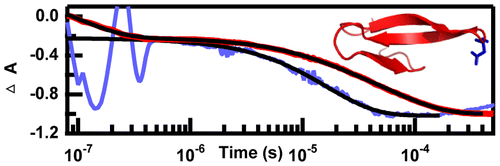
The role of electrostatic interactions in folding of β-proteins
DOI: 10.1021/jacs.5b13201
PMID: 26750867
[5] Davis, C.M.; Dyer, R.B. “The role of electrostatic interactions in folding of β-proteins,” J. Am. Chem. Soc. 2016, 138 (4), 1456-1464.
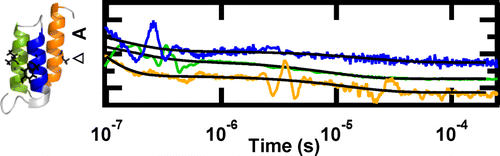
Fast helix formation in the B domain of protein A revealed by site-specific infrared probes
DOI: 10.1021/acs.biochem.5b00037
PMID: 25706439
[4] Davis, C.M.; Cooper, A.K.; Dyer, R.B. “Fast helix formation in the B domain of protein A revealed by site-specific infrared probes,” Biochemistry 2015, 54 (9), 1758-1766.
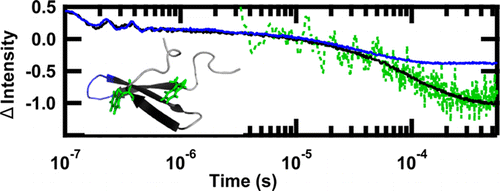
WW Domain folding complexity revealed by infrared spectroscopy
DOI: 10.1021/bi500556h
PMID: 25121968
[3] Davis, C.M.; Dyer, R. B. “WW Domain folding complexity revealed by infrared spectroscopy,” Biochemistry 2014, 53 (34), 5476-5484.
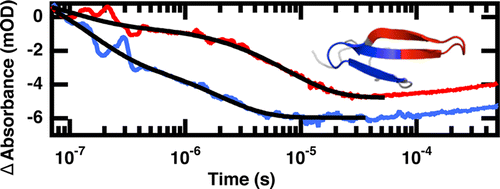
Dynamics of an ultrafast folding subdomain in the context of a larger protein fold
DOI: 10.1021/ja409608r
PMID: 24320936
[2] Davis, C.M.; Dyer, R. B. “Dynamics of an ultrafast folding subdomain in the context of a larger protein fold,” J. Am. Chem. Soc. 2013, 135 (51), 19260-19267.
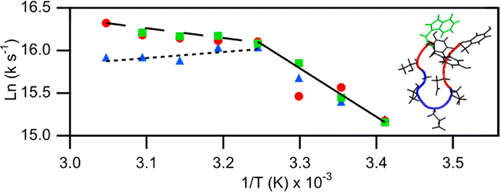
Raising the speed limit for β-hairpin formation
DOI: 10.1021/ja3046734
PMID: 22873643
[1] Davis, C.M.; Xiao, S.; Raleigh, D.P.*; Dyer, R. B.* “Raising the speed limit for β-hairpin formation,” J. Am. Chem. Soc. 2012, 134 (35), 14476-14482.